Huawei, SMIC, and Future of Export Controls: SemiAnalysis and Fabricated Knowledge in Conversation
“There isn’t just that forward-thinking of where the ball is going to; instead, it’s constantly looking back at where the ball was.”
Huawei’s breakthrough Kirin 9000S chip — what is it, why is it a big deal, and what should America do about it? Last week, Dylan Patel of SemiAnalysis and Doug O’Laughlin of Fabricated Knowledge joined ChinaTalk for an emergency episode. We discuss:
Why this new smartphone chip is so impressive;
What it takes to go from smartphone chips to AI hardware accelerators at scale, and why doing so may be easier than policymakers currently think;
What we know about SMIC’s yield;
Economic and military knock-on effects of a SMIC capable of fabbing A100-equivalent AI chips;
How global semicap and software firms helped build the Mate 60 Pro;
How advanced packaging and other uncontrolled technologies on the industry roadmap make the EUV limitation less of a bulwark to ensuring non-Chinese fabs maintain their edge over time;
Steps that BIS and US allies could take to significantly impair continued Chinese leading edge chip advancement, and why it will take more than just squeezing on one chokepoint to make a sustained impact.
Huawei’s Success Story
Jordan Schneider: To start off, what is the Kirin 9000s chip, and why is its so impressive?
Dylan Patel: The Kirin 9000s chip is Huawei’s newest smartphone chip. On the surface, it’s not really that big of a deal: it doesn’t break any of the benchmarks; it’s not really for anything that’s strategically important. It’s just a smartphone chip.
But when you dig a little bit deeper, it’s actually an amazing breakthrough from multiple angles, all the way from design to manufacturing. From the design standpoint, Huawei designed a 5G modem, which they’ve done in the past; they had the best 5G modem before the bans came down a few years ago. Furthermore, it has a custom CPU and GPU.
This is pretty impressive because Huawei didn’t have a custom CPU or GPU in the past, but now they do, showing that they’ve not only retained their design chops after US sanctions — they’ve in fact increased them.
Furthermore, the manufacturing is on SMIC’s N+2 process technology. SMIC, for some reason, has stopped naming their process technologies. Instead, it just calls it N+1, N+2, … N being 14 nanometer, N+1 being about 10-nanometer and N+2 being 7-nanometer, more or less in terms of the process geometries. This is a very big deal because China now has a domestic 7-nanometer process technology, and it’s in a real product. N+1 was a Bitcoin mining chip, so it didn’t really have a broad range of IPs, but this is a 7-nanometer and a smartphone chip, which kind of has every kind of IP possible. It has a cellular modem, AI IP from the neural processing engine, image signal processing, various CPUs/GPUs, storage, media encoding … all this sort of stuff on a smartphone SOC.
So they’ve demonstrated a whole host of IP on a leading-edge process technology that’s on par with the best of what Intel is still shipping today, which is what America has. Intel’s best process technology that I can go out and buy today is an Intel 7. And what’s the best process technology from China that I could buy today? It’s a SMIC 7. So while Intel is supposed to be releasing chips with laptops coming this year with their 4, 7 is what both America and China are on today. It’s on par, if you want to look at it from that angle. So that’s extremely important.
Jordan Schneider: And just to be clear, these are from two companies — Huawei and SMIC — that are on the Entity List. So it’s not just that you’re faced with the October 7 regulations, but you have to go above and beyond and apply for licenses basically every time you want to do anything with an American company.
Dylan Patel: Well, those licenses are practically irrelevant because there’s a whole host of shell companies that you can spawn. If you talk to Lam Research — an equipment company for manufacturing semiconductors — and you ask them, “Hey, what’s the sustainability of lagging-edge semiconductor equipment?” they’ll tell you, “Oh yeah, it’s great. There are some companies that have been put on the Entity List, but there are ten new companies that came out, and they’re building fabs.” And it’s like, “Oh, come on.” I mean, some of them are new companies building fabs for sure, but some of them are just being routed to SMIC — and in some cases they’re just being granted licenses to ship to SMIC. Licenses are handed out like candy, and the Entity List is not really bulletproof. I don’t really see how that’s relevant.
Doug O’Laughlin: The tools that work for the lagging edge can often be used for the leading edge. The thing that we have obviously clamped down the hardest on is EUV: no EUV tools are shipped into China. But, for example, a metal deposition tool that’s being used for bigger pitches can also be used for lower-level pitches. The line there is extremely gray, and I don’t think that the export controls really consider the fact that a lot of these lagging-edge tools can mostly be used on the leading edge. The precision is not exactly constrained by anything other than the pattern, meaning the lithography. The lithography is what defines the pitches, but all the tools can probably work with that level of precision.
There’s this giant loophole in the fact that most of the tools that are being sold to the lagging edge can obviously be used on the leading edge.
Also, I think that people have underestimated or underappreciated the Kirin SMIC chip because this 7-nanometer technology density and performance is pretty on par with Intel. This was originally Intel 10 that has been renamed to Intel 7. So you’re telling me that the giant hiccup that Intel missed for years is pretty much what SMIC managed to completely nail, while the technology has been restricted from them? I think that the competitive dynamic there is really underappreciated because they managed to pull off what Intel could not with more restrictions and in a shorter timeframe.
Dylan Patel: Although, I would argue that no equipment is restricted that wasn’t used in Intel’s 10-nanometer/7-nanometer process node. Intel 7 uses the NXE:1980I, which is a specific lithography tool from ASML. That tool is not restricted from being shipped into China. Well, if I can get any tool I want from just a random shell company in China or just get it shipped to me because licenses are handed out like candy, what am I even being restricted on? There are restrictions around EDA, which is software for helping design chips, but that only applies to 3-nanometer chips, not 7-nanometer chips. So again, there’s nothing being restricted.
There are restrictions around a whole host of things, but none of them applies to what SMIC and Huawei are doing, even though the October 7 regulation was very clear in saying, “No company should be assisting Chinese companies with below 14-nanometer using American technology.” And yet they’re all doing it because there are massive loopholes in the regulation.
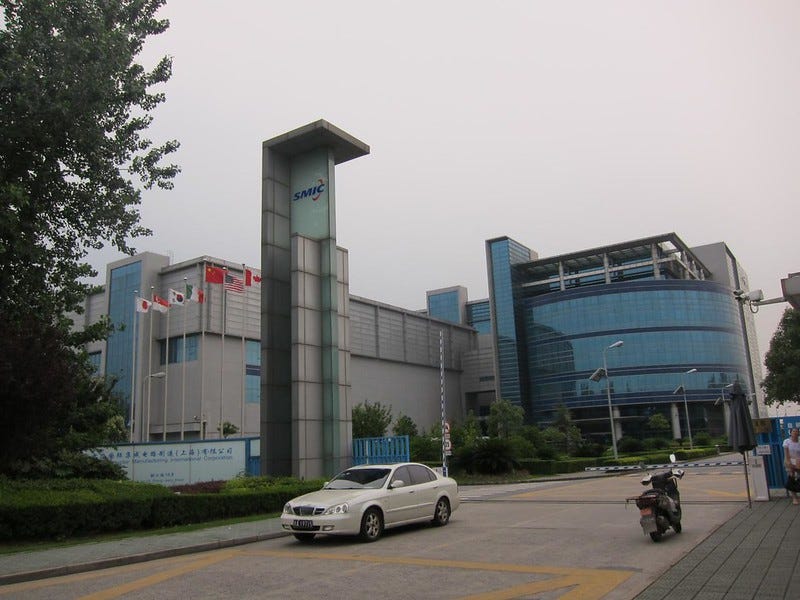
Why Chinese Firms Can Still Get Advanced Foreign Tools
Jordan Schneider: This is one of your spicier claims in your recent post on SemiAnalysis — the fact that this is being done with blindfold negligence or active support of all these foreign players in the US, Japan, Germany, and so on. So is it plausible that the KLAS and Tokyo Electrons of the world would not know what their machines are being used for? The machines need ongoing support: don’t you go into the fabs and look at it and fix it, and understand what wafers are coming out on the other side?
Doug O’Laughlin: A lot of things are like, “You buy this tool, there are definitely some process engineers who know what’s going on — but if you get the latest and greatest you’re going to have to do a lot of co-work with the tool company.” And that’s where Applied Global Services (AGS) — which is the largest service company — comes in. They come in and they help you figure out how to use your tool for the best application.
Dylan Patel: Also do continual maintenance for the tools: constant upgrades, spares, make sure your uptime is very high … Applied Materials pretty much attaches an AGS service contract with most of the tools they service and sell. They brag about how high the attach rate is, how it helps their customers, and how it’s so profitable for them. And at the same time, they’re doing this in China.
So yeah, they should be seeing this — but never attribute malice when ignorance is possible. Maybe it’s just ignorance, but in many cases you know exactly what the tool is doing. “Hey, we’re having problems depositing this 36-nanometer copper metal line. What’s going on? Can you help us tune the tool?” It’s like, “Well, a 36-nanometer copper metal line is what TSMC 7-nanometer uses. There’s no 14-nanometer that uses 36-nanometer copper lines.” So they should be able to tell — maybe it’s just malice, maybe it’s ignorance.
Doug O’Laughlin: Yeah, maybe they’re just answering whoever’s working on it. Or for example, Applied might have a domestic Chinese service force which does all the servicing — and then they interact or they work with the global AGS. I don’t know. It’s really hard to know because there are thousands of steps in making a semiconductor. And I think ASML is weirdly, we’ll say, negligent here: the DUV tools are really interesting as a concept — the difference between a 1980 and a 2000 one is essentially some overlay tools.
But my question is: if ASML is selling and servicing spares and retrogrades, can they not retrograde the 1980 tool with a better overlay tool? Or if there’s not an entire industry devoted in China to improving overlay in DUV, it just seems like an obvious loophole. There have been so many obvious loopholes that you could drive a bus through — people have just been watching and waiting, and then twelve months later you’re like, “Oh, SMIC 7, how did this possibly happen?”
Jordan Schneider:
There is no way that China today could be making 7-nanometer, 14-nanometer, or even 28-nanometer without Western tooling, equipment, and support.
And I think this was illustrated really dramatically when I walked around SEMICON with you guys. There must’ve been 600 companies there, and every single one of them, in some way, shape, or form is involved. And that's why they’re there. They’re real companies. Some are large, some are small — but they’re in this ecosystem. Domestic Chinese players were really a pretty small percentage — basically only four. And none of them is proffering end-to-end solutions for manufacturing lagging-edge semiconductors, much less something like a 14- or a 7-nanometer. So the fact that these chips are coming out at all in scale means that there is a ton of global support which is going into enabling the production and yield.
Doug O’Laughlin: Domestic Chinese players have only ever been able to carve out extremely small parts of the process of its leading edge — or they carve out much older lagging edge, with much lower precision. For example, SMEE, the Chinese lithography company, essentially has nothing to do with lithography in the way that we think about it. Most of the lithography that’s happening there is for advanced packaging and printing litho patterns onto a PCB — very different precision, very different product.
I think the other part that is underappreciated here: there are a lot of Japanese and Korean firms, and that might be the way that you can get around this as well, to a certain extent. For example, Tokyo Electron has a lot of N10 solutions. Tokyo Electron often can’t compete with Applied on a head-to-head basis for most of the tools — there are many times when they’re number two and number three — but they do have a full solution. So that’s one of the ways that they can be getting tools. I think another big thing is Korean tool companies, which are often mostly memory-captive.
Those are some incremental ways, but at the end of the day, the American semicap companies really are three of the big five companies. And at the end of the day, they really drive a lot of the boat, especially the random steps. Lithography is ASML. Tokyo is cleaning. But everything else in the middle oftentimes comes to the American semicap. Clearly there’s American help.
Jordan Schneider: The import substitution strategy is a multi-decade-old one. If you take the pieces of the Jenga puzzle out, you will not find other pieces of that, however much you want to spend and however much industrial espionage you want to do. This is an extremely difficult — if not probably impossible — thing for the Chinese broader ecosystem to be able to replicate domestically even with the help of Japanese and Korean firms.
Dylan Patel: I like that analogy a lot — because essentially what you’re doing is you’re giving them a puzzle, you’re taking one piece out, and saying, “You’re not allowed to have that one piece of the puzzle.” It’s like, “Okay, well, we can make that piece of the puzzle, and it’ll look exactly like the original puzzle.” But if you take out a hundred pieces of the puzzle and it’s a 200-piece puzzle, then that’s impossible; it takes way too much engineering, way too much time, way too much capital. But if there are just a few pieces taken out — which is what’s happened today — then the puzzle can be completed. The investment is large, but it’s not insurmountable, and the cost of doing it is not insurmountable.
Doug O’Laughlin: The proof is in the pudding. There have been some really good charts of American exports into China for semicap. The chart has gone parable essentially. It is the single biggest difference between what we thought WFE (wafer fab equipment) would be, year-to-date. And what it actually has ended up being has been just semicap demand. Demand is at the highest on a monthly basis it’s ever been. I know they’re making a lot of lagging-edge fabs, but there is a 0% chance that a meaningful amount of those tools are not being used for SMIC 7.
Dylan Patel: The other thing I would point out is that Applied Materials has this entire business unit called ICAPS, and that’s for this trailing edge stuff: IOT, communication, sensors, all this sort of stuff. And that business unit has basically bailed out the rest of the company as the world economy has slowed and people have cut back.
TSMC cut $4 billion of spending this year from what their original plan was. Intel cut a little bit silently; Samsung cut. These major non-Chinese companies cut. Applied Materials is doing quite well because of this ICAPS business unit. Now, some of that is going internationally, but a lot of that is China. And if you look at the percentage of sales, it’s very clear that this ICAPS business unit is shipping a ton of tools there. It’s like that jigsaw puzzle: we’ve taken out one piece, and they can clearly route them around.
How to Guesstimate SMIC’s 7nm Yield
Jordan Schneider: 14-nanometer was apparently the line that the Biden administration tried to set. We’re now at 7, and we’re at scale with pretty promising yield. Dylan, can you talk us through the data points that you used to come to the conclusion that this isn’t just a one-off thing, but actually something that they’ll be able to manufacture at scale and relatively economically?
Dylan Patel: With any manufacturing process with tons of steps, maybe you can get one or two outright with a lot of money thrown in. And that’s been the argument that certain pundits have been putting out: “Hey, SMIC has really, really bad yield.” I don’t doubt they have worse yield than TSMC, Samsung, or Intel; they just got here. That’s how process technology works. You start with a very simple chip — smartphone chips, small smartphone chips — and then you move up and up and up until you finally have these massive data center-scale AI chips and CPUs that are used in data-center servers. The thing that some pundits have been saying is that the yield is very low — and I don’t know if that’s really the case.
One, there’s a source that said the D0 is about twice what TSMC has, but TSMC is the gold standard. D0 is the number of defects you have per square centimeter, and that’s just how many transistors don’t work. So that metric was leaked out, but that’s hearsay — hard to say this is good or bad.
The other thing you can do is look at the reverse engineering analysis that TechInsights has done. If you look at the structures, how the various metal layers look and how the transistors look is not horrible. When you have bad yield, your metal layers might be more wavy, but they’re not wavy. They look pretty straight. And this is qualitative. FinFETs — they’re pretty straight, they’re not super wavy. There are not many shorts or things like that that you can see.
And then the last data point is from people who have been posting benchmarks. This is the hottest phone in the world to post a benchmark on — even hotter than the new iPhone release. A year and a half ago, Qualcomm switched to a new Samsung process node: Samsung 4, for their SAG 1 smartphone chip. It was a brand-new process node from Samsung. It was difficult for them to do, and the yield wasn’t good — that was reported by many outlets. And then once the chip came out, you could see a variation as much as 10% between two phones with the exact same chip. And why is that? There are a couple aspects of yield. Yield can be, “Hey, it doesn’t work at all. This transistor doesn’t work. This contact shorted itself. This metal layer bridges to another metal layer. It just doesn’t work.” But then there’s another aspect — “Hey, if you look at the features, they’re wavy. That increases resistance slightly. That increases the switching time for a transistor.” So you end up with what’s called parametric yield, which is,
“Hey, the chip still works, but how many actually reach the targets? Can it reach this frequency at X power?”
That’s this idea of parametric yield. That’s really where most yield losses go in the semiconductor manufacturing industry. It’s not, “Hey, the chip doesn’t work at all.” It’s like, “It works, but a handful of transistors can’t switch fast.” You can’t get the parameters you want out of the chip.
When you have a bad yield, a lot of times you do what’s called bending. You take chips that you’ve manufactured, you test them all, and you say, “Hey, some are the best. These are great, some are good, some are okay, some are bad.” Then you set a line — you’re like, “Hey, for the chip, we’ll accept great and good.” That’s where you define the product. And the interesting thing is if you have bad yield, generally your product varies a lot more. And this even applies to Intel’s first 10-nanometer I5 laptop CPUs. There’s a lot of variance in that Qualcomm chip that I was referring to earlier.
In the benchmarks that I’ve seen online for Kirin 9000S — and there needs to be a lot more testing done — there doesn’t seem to be a ton of device-to-device variation, which implies that their parametric yield is actually pretty good, which would imply their real yield is good. Now, all of these are soft, but I don’t believe for a moment — unlike some pundits who are trying to downplay the significance of it — that this chip has a 10% yield.
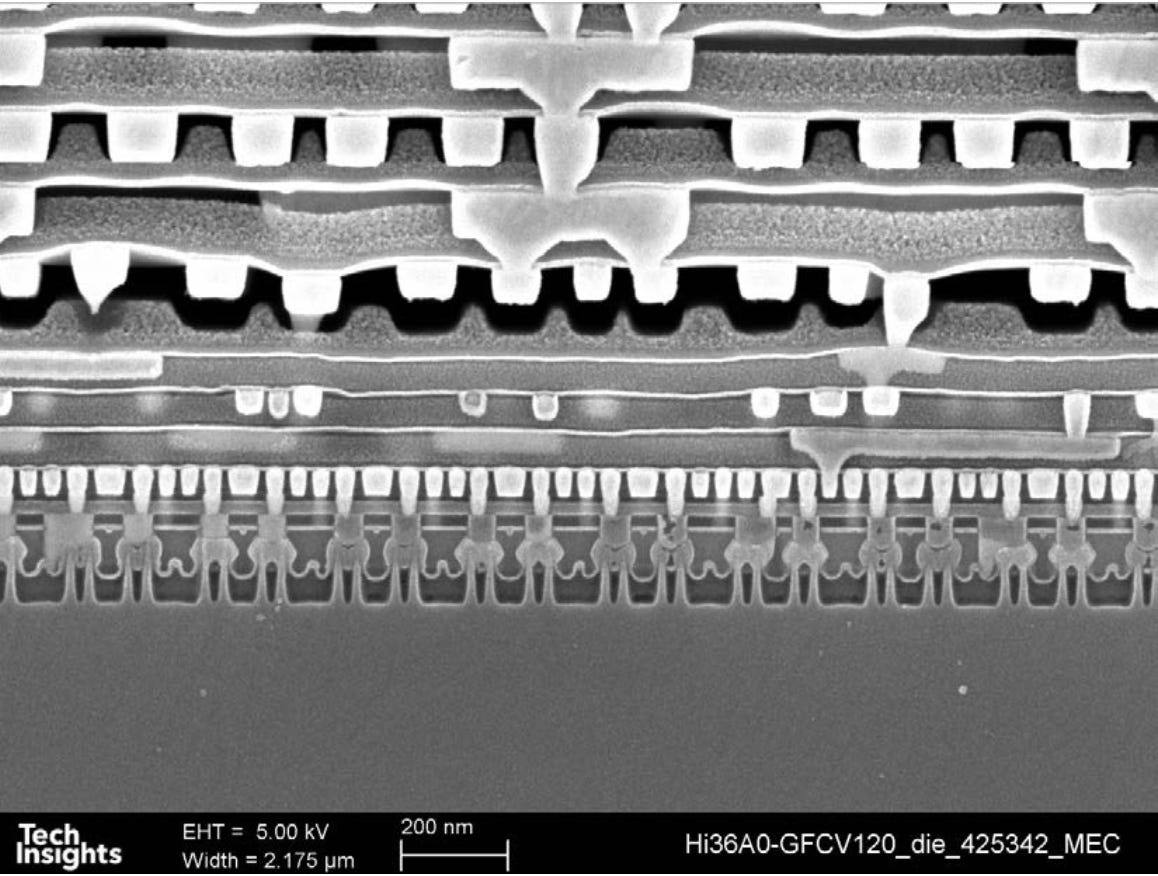
Subscribers get access to our full conversation. We get into:
What it takes to go from smartphone chips to AI hardware accelerators at scale, and why doing so may be easier than policymakers currently think;
Economic and military knock-on effects of a SMIC capable of fabbing A100-equivalent AI chips;
How advanced packaging and other uncontrolled technologies on the industry roadmap make the EUV limitation less of a bulwark to ensuring non-Chinese fabs maintain their edge over time;
Steps that BIS and US allies could take to significantly impair continued Chinese leading-edge chip advancement, and why it will take more than just squeezing on one chokepoint to make a sustained impact;
What policy signals are coming out of BYD’s European travails;
Why tradeoffs between electrification and geopolitics are emerging;
And how BIS can tighten export controls without starting a war.